Scientists Discover Islands Of Magnetic Stability That Could Unlock Fusion Power
Tokamak's biggest weakness could soon be fixed.
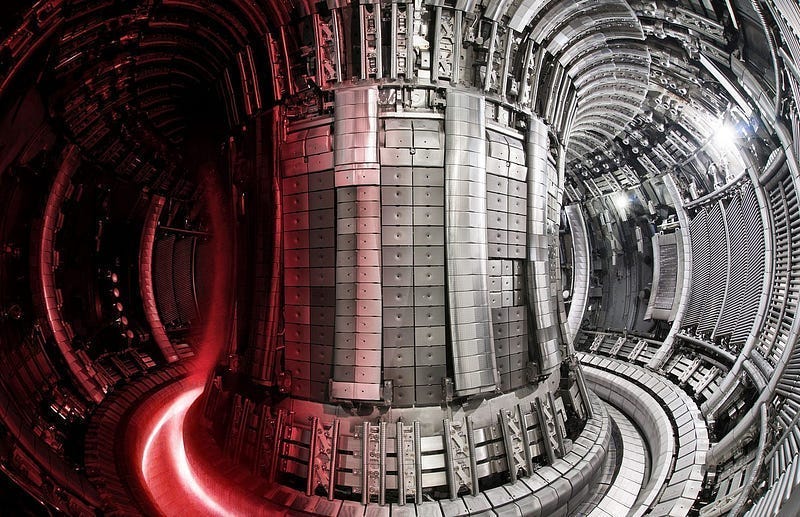
You may have heard of a little thing called nuclear fusion. It powers the Sun and could be the ultimate clean energy. Theoretically, we could harness it to produce near-limitless amounts of on-demand energy with zero carbon emissions and practically no nuclear waste. But there is a problem: the fusion reactors we currently have use more energy to create fusion than they get out of the fusion reactions, making it a useless energy source. Even the most promising and refined reactor design, known as a tokamak, struggles with this issue. One of the significant obstructions stopping tokamaks from overcoming this issue is periodic plasma instabilities called edge-localised modes (ELMs). These instabilities in the edge of the reactor’s plasma fuel result in a considerable amount of energy leaking into the walls of the reaction chamber. Not only does this cause the fusion reactions to fizzle out, but it can severely damage the tokamak itself. However, Physicists from Germany and the US have discovered a brilliant way to solve this problem by combining two emerging plasma stabilisation techniques. So, the question has to be asked: could this unlock fusion power?
As always, when discussing fusion, let’s quickly recap what it is and why we are struggling to bring this utopian technology to fruition.
As I said, nuclear fusion is the process that powers the Sun. In its core, the temperatures are so high, and the pressure is so intense, that hydrogen atoms have enough kinetic energy to overcome the repulsive force that keeps them separate and fuse into a single larger helium atom when they collide. Helium weighs slightly less than two atoms of hydrogen, so there is a bit of spare mass floating around in the form of subatomic particles. These particles can’t physically exist without being attached to protons or neutrons, so they turn into energy and radiate out. Now, if you paid attention in physics class, you’d remember that Einstein’s famous equation E=MC² means that a tiny bit of matter is equal to an utterly vast amount of energy!
As such, a single kilogram of hydrogen can produce 177,717 MWh of energy through fusion, or 7.9 times more than a kilogram of pure uranium-235 produces through fission or 14.8 million times more energy than a kilogram of gasoline through combustion! But, unlike uranium or gasoline, fusion doesn’t produce any radioactive waste or climate-destroying emissions. The only by-product is valuable non-radioactive helium gas. As such, nuclear fusion is arguably the perfect energy source.
We have several fusion reactor designs, but the tokamak is the most promising. They are comprised of a doughnut-shaped reaction chamber surrounded by superconducting electromagnets. During operation, hydrogen plasma is injected into the reaction chamber. As hydrogen plasma is conductive, it can be pushed, pulled and heated by intense magnetic fields. As such, the superconducting electromagnets heat and squeeze the hydrogen plasma to the point where fusion occurs. This is where our plasma instabilities, also known as edge-localised modes (ELMs), happen.
ELMs are pretty complex, so I will try to explain them as simply as possible. At the plasma’s edge, the density gradient is massive, which can cause ripple-like instabilities on the surface of the plasma. These instabilities interfere with how the plasma interacts with the magnetic field and can periodically relax the squeezing force felt by the plasma. When this happens, it is like popping a balloon, and the energy within the plasma is released in the form of vast amounts of heat and an enormous amount of high-energy particles.
A single ELM can cause the plasma to lose up to 20% of its energy! This makes the tokamak less efficient, making net gain in energy even more challenging to reach. In fact, ELMs get more frequent when tokamaks operate at higher powers, and these higher powers are crucial for creating enough fusion to make achieving a net gain in energy possible. In other words, without finding a way to stop ELMs, a tokamak might never be able to be used as an energy source.
This is where our brilliant scientists come in.
They discovered that by combining two methods that previously struggled to suppress ELMs, namely resonant magnetic perturbations (RMPs) and electron cyclotron current drive (ECCD), they could create a new plasma stabilisation method that stops them in their track! Before we try to understand this breakthrough, we first need to quickly learn how these two plasma stabilisation methods work.
Resonant magnetic perturbations (RMPs) create ripples in the tokamak’s magnetic field that, in turn, create closed loops of magnetic fields inside the plasma. These closed loops are commonly called magnetic islands, and in theory, they can redistribute the pressure within the plasma, reducing the plasma density gradient and, therefore, stopping ELMs. However, only if the magnetic islands are precisely the correct size. Otherwise, they can destabilise the plasma even more, creating even more ELMs!
Electron cyclotron current drive (ECCD) is very different. They inject the plasma with high-frequency microwaves, which causes electrons to spiral in the direction of the confining magnetic field lines, generating local currents which run parallel to the field lines. These moving electrons create their own magnetic field (as all moving electrons do), which, like the RMP method, redistributes the pressure within the plasma, making ELMs less likely to happen.
These scientists ran a computer simulation to test how these two methods could be used together. Firstly, they found that the ECCD lowered the energy required to create RMPs. As efficiency is the name of the game here, that alone is potentially a giant leap forward. However, they also found that they could use ECCD to have precise control over the size of the RMPs. By changing the direction of the ECCD in the plasma, they could increase or decrease the size of the RMPs, allowing them to fine-tune their size and completely eliminate those pesky edge-localised modes (ELMs).
As such, this novel plasma stability method can enable tokamaks to run more efficiently and at way higher power levels without destroying themselves or incurring huge losses. This could even help tokamaks create “burning plasma”, where energy from fusion is retained within the plasma and sets off more fusion in a self-sustained burn, a crucial step in reaching a net gain in energy that tokamaks have yet to reach. But this is in theory; what about practice? Well, these results are being used to assess how to run ITER, the world’s largest and most powerful tokamak, currently under construction in France, and due for its first fusion experiments to start in 2025. ITER is purported to be able to produce ten times the energy it takes to run it from fusion! This is if its plasma is stable enough. So, if these simulations can be replicated in ITER, fusion power will finally be unlocked! But we might have to wait. It could still be another decade before ITER is ready to test this hybrid plasma stabilisation method. But, I think you will agree, it will be worth the wait.
Thanks for reading! Content like this doesn’t happen without your support. So, if you want to see more like this, don’t forget to Subscribe and follow me on BlueSky or X and help get the word out by hitting the share button below.
Sources: Physics World, Nature, SciTechDaily, OSTI, Nature, Planet Earth & Beyond