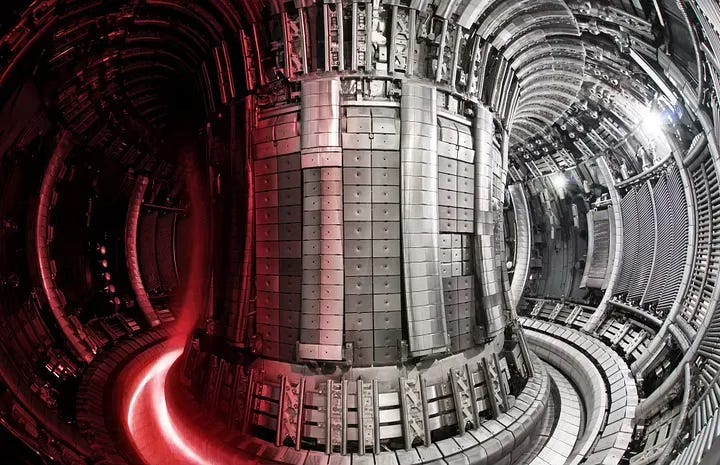
Nuclear fusion power could revolutionise the world. That isn’t hyperbole. In theory, fusing a few tonnes of hydrogen can create enough power to run the largest countries on Earth for an entire year, with the only by product being helium. Not only would such technology solve the climate crisis, but it would also enable us to access far more energy than we have ever had. But, in practice, things are a little tricky. None of the fusion reactors we have ever built can produce more energy than it takes to run them, and they are so expensive that, even if they did produce a net gain in energy, they wouldn’t be a viable power source. But all of that might be about to change thanks to MIT and Commonwealth Fusion Systems (CFs) and their insane high-temperature superconducting electromagnets.
To understand the impact of this brilliant breakthrough, we first need to understand what fusion is and how we create nuclear fusion in a tokamak.
Nuclear fusion is the process that powers the Sun. In its core, the temperatures are so high, and the pressure is so intense, that hydrogen atoms have enough kinetic energy to overcome the repulsive force that keeps them separate and fuse into a single larger helium atom when they collide. Helium weighs slightly less than two atoms of hydrogen, so there is a bit of spare mass floating around in the form of subatomic particles. These particles can’t physically exist without being attached to protons or neutrons, so they turn into energy and radiate out. Now, if you paid attention in physics class, you’d remember that Einstein’s famous equation E=MC² means that a tiny bit of matter is equal to an utterly vast amount of energy! As such, a single kg of hydrogen can produce 177,717 MWh of energy through fusion, or 7.9 times more than a kg of pure uranium-235 produces through fission or 14.8 million times more energy than a kg of gasoline through combustion!
This insane energy density, combined with the fact that the only by product of the reaction is helium, is why scientists and engineers have been hard at work for decades trying to replicate this process on Earth.
This is where the tokamak comes in. The tokamak is one of the most promising fusion reactor designs we have. It consists of a doughnut-shaped reaction chamber filled with hydrogen and surrounded by incredibly strong superconducting electromagnets. To create fusion, the hydrogen is heated to the point it becomes a plasma. Hydrogen plasma interacts strongly with magnetic fields, so at this point, the electromagnets take over. They heat the hydrogen up even more, in a similar way to how an induction stove heats up your pans, and starts squeezing the plasma. This creates atomic collisions within the plasma with enough kinetic energy to create fusion. Most tokamaks operational today are experimental, so they let the energy produced through fusion radiate away, but the idea is that eventually, this energy would be captured and turned into electricity through flash heating water into steam to drive turbines.
In theory, tokamaks are great. They can run continually and be refuelled on the fly, making them an ideal candidate for a usable fusion power source. But they are currently inefficient. The highest-ever Q factor reached by a tokamak was only 0.67 by JET in 1997. In other words, for every kWh it took to run the reactor, it only produced 0.67 kWh in fusion energy, or a net loss of 33%.
This is where MIT’s and CFS’s breakthrough comes in.
Three years ago, they successfully tested a new type of high-temperature superconducting electromagnet for CFS’s upcoming tokamak reactor SPARC. Superconducting is when a material reaches zero electrical resistance, typically when they are cooled to almost absolute zero, and this process is the only way to make electromagnets powerful enough for tokamaks. Well, the two have now published several papers confirming their results. This superconducting magnet produced a record-breakingly strong magnetic field of 20 Tesla at a temperature of 20 degrees Kelvin (-423.67 F / -253.15 C). For some sense of scale here, ITER, the world’s largest and most capable tokamak currently under construction, has electromagnets that produce a magnetic field of 11.8 Tesla at 4.5 degrees Kelvin (-451.57 F / -268.65 C).
How did they achieve this? Well, thanks to their high-temperature superconductor material known as REBCO, they don’t need to insulate the superconducting wires of the electromagnet. Instead, the much higher conductivity of REBCO keeps the wires insulated from either other without the need for insulation. Eliminating the insulation dramatically simplifies the fabrication processes and leaves more room for other elements, such as more cooling or more structure for strength, while also allowing them to operate it at lower voltages. In other words, this electromagnet is not only super strong but takes less energy to operate and is far easier and cheaper to build than the electromagnets we currently use in tokamaks.
So, why does this matter?
Well, remember I mentioned SPARC? The Tokamak CFS is currently building. Well, according to physicists, a magnetic field of 20 Tesla is needed for it to produce a net output of power. Not only does this electromagnet meet that requirement, but its high-temperature nature, low voltage operation and ability to cool it far more efficiently means that it will take less energy to run, making it easier to produce a net output or increasing the power it can output. But these magnets are also incredibly cheap compared to those we currently use. One physicist on the team stated that “overnight, it basically changed the cost per watt of a fusion reactor by a factor of almost 40” and that “Now fusion has a chance of being economical.”
So, will this breakthrough lead to super-affordable fusion power stations capable of delivering vast amounts of power to the grid? We will have to see. SPARC isn’t going to produce its first fusion reactions until next year, and it won’t start full operations until the early 2030s. If these tests go well, then MIT and CFS will have unlocked the most revolutionary technology ever created by humanity. But even in a best-case scenario, this technology is still decades away from making it out of the lab, and into the world.
Thanks for reading! Content like this doesn’t happen without your support. So, if you want to see more like this, don’t forget to Subscribe and follow me on BlueSky or X and help get the word out by hitting the share button below.